Weizmann (Israël) analyse les éléments émis par une étoile inhabituelle juste avant l'explosion
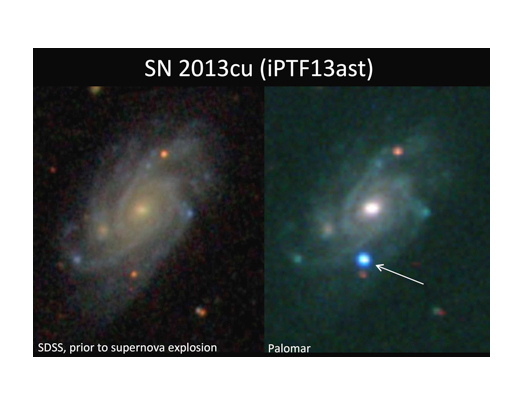
[:fr]Lorsqu’une supernova (explosion d’une étoile éloignée) a été découverte l’an dernier, les astrophysiciens du monde entier se sont précipités pour observer ce feu d’artifice à l’aide de télescopes. Dans un dernier éclat spectaculaire, l’étoile – rare car ayant une masse plus de dix fois celle de notre soleil –donne des informations sur la vie de ces corps cosmiques fascinants, et permet de se faire une image de la formation des éléments lourds dans l’univers.
Pour comprendre l’étoile qui a produit la supernova, les chercheurs de Weizmann ont identifié la composition des éléments rejetés juste avant le début de l’explosion.
Le Pr Avishay Gal-Yam, du département de Physique des particules et d’astrophysique de l’Institut Weizmann, explique que « l’étoile peut être identifiée par la proportion d’éléments tels que le carbone, l’oxygène et l’azote détectés dans la matière rejetée dans l’espace. Ces éléments sont créés dans la fusion nucléaire qui alimente les étoiles en énergie. Dans notre soleil, les atomes d’hydrogène (atome le plus léger) fusionnent pour donner naissance à un atome d’hélium – et s’arrêtent là ; mais dans les étoiles massives et plus chaudes, la fusion continue : les atomes d’hélium s’unissent pour former des éléments de plus en plus lourds – jusqu’à devenir des atomes de fer ».
Selon les chercheurs de Weizmann, ces étoiles sont organisées en couches, comme les oignons : les éléments les plus lourds, par exemple le fer, se trouvent dans leur centre, tandis que les plus légers forment les couches externes. En bordure des étoiles, les vents stellaires soufflent les matériaux des couches externes vers l’espace. Dans les étoiles comme celle qui a explosé, le vent est si violent qu’il peut projeter au loin, tous les 10 000 ans, une masse égale à celle de notre soleil. A un certain moment de la vie de l’étoile, l’hydrogène léger qui forme sa couche extérieure s’épuise, et elle commence à rejeter l’hélium, l’oxygène, le carbone et l’azote.
Sous la surface se trouve une couche réunissant hydrogène, hélium et les éléments plus lourds.
Cette couche doit être assez élevée pour contenir de l’hydrogène, mais aussi assez chaude pour produire les températures extrêmes nécessaires à la fusion nucléaire. Les chercheurs s’intéressent à cette couche car c’est là que se forme l’azote. Contrairement au carbone qui contient six protons (par fusion de trois atomes d’hélium), et à l’oxygène, qui en a huit (provenant de quatre atomes d’hélium), l’azote a sept protons, un nombre impair ! Ceci signifie qu’il doit être le résultat d’une fusion entre des atomes pairs et impairs, par exemple trois d’héliums et un d’hydrogène. Par conséquent, si l’on mesure les quantités d’azote, on pourra découvrir ce qui se trouve sous la surface de ces étoiles.
Lorsque le vent chasse les couches extérieures de l’étoile, son cœur continue à amasser du fer jusqu’à ce qu’il devienne si lourd qu’il perd sa stabilité. A ce point, le cœur s’effondre dans un mouvement soudain et violent, rejetant les couches externes pour produire la brillante supernova que nous observons. On ne peut observer les éléments éjectés dans le vent stellaire, juste avant l’explosion, que dans un espace de temps limité, jusqu’à un jour environ après la déflagration finale. Cela est dû au fait que la radiation intense produite par le choc de l’explosion arrache les électrons de leurs atomes. Les télescopes équipés de spectrographes dirigés vers la supernova peuvent saisir les spectres des éléments, c’est-à-dire la lumière émise lorsque les électrons s’unissent à nouveau aux atomes.
Mais ils doivent faire ces observations rapidement, avant que les débris de l’explosion (qui se dispersent rapidement) balayent jusqu’aux restes les plus petits du vent et effacent cette dernière trace de l’étoile mourante. La course pour observer les spectres du vent de la supernova a commencé avec les télescopes robotiques de l’observatoire du Mont Palomar, en Californie, qui font partie du programme iPTF dirigé par le professeur Shri Kulkarni, de Caltech (California Institute of Technology). Ces télescopes robotiques sont programmés pour trouver des événements transitoires – des changements soudains dans le ciel nocturne qui pourraient être de nouvelles supernovae – et alerter les membres du groupe.
A l’autre bout du monde, le Dr Iair Arcavi, à l’époque doctorant dans le groupe du Pr Gal-Yam, a appris la nouvelle. A l’heure où les chercheurs américains dormaient, il a analysé les données, a compris ce qu’elles signifiaient, et a pris contact avec le Dr Assaf Horesh, aujourd’hui à l’Institut Weizmann, mais à l’époque postdoctorant à Caltech. Le Dr Horesh a fait alors des observations spectroscopiques à l’observatoire Keck, à Hawaï, qui est plus à l’ouest que Palomar, ce qui lui a permis de continuer sa recherche lorsqu’il faisait déjà jour en Californie, alors qu’à Hawaï c’était encore la nuit. Grâce à la rapidité de son travail, il a réussi à enregistrer les spectres d’émission des matières emportées par le vent à peine quinze heures après que l’étoile a explosé.
L’étude des données obtenues par le Pr Gal-Yam, et les docteurs Arcavi et Horesh, et leurs collègues, a permis de confirmer les spectres enregistrés et a montré que l’étoile qui avait explosé avait bien un vent riche en azote, semblable à ceux des étoiles Wolf-Rayet que l’on connaît dans notre galaxie. C’est la première fois, selon le Pr Gal-Yam, que ces observations sont faites. Maintenant que le groupe a montré que la combinaison d’une organisation mondiale efficace avec la mobilisation de télescopes un peu partout dans le monde peut servir à capturer des événements éphémères, ses membres espèrent qu’ils auront la possibilité de voir d’autres explosions de jeunes supernovae.
Selon le Pr Gal-Yam, il est important de comprendre comment vivent et meurent ces étoiles, et pas seulement parce qu’elles nous donnent des informations sur le fonctionnement de l’univers. Il ajoute : « Les éléments plus lourds, ceux qui ont une masse plus grande que celle de l’hélium, sont créé dans les fourneaux de fusion des grandes étoiles et sont dispersés par les explosions de supernovae. Un si grand nombre de questions – sur les origines et l’abondance relative des différents éléments – sont soulevées par ces processus qui ont eu lieu un peu partout dans le cosmos. »
Publication dans Nature, 22 mail 2014.
[:en] When a supernova – the explosion of a distant star – was discovered last year, astrophysicists, with the help of telescopes around the globe, rushed to observe the fireworks. In its dramatic dying flares, this star – a rare type over 10 times the mass of our sun – can tell us something about the life of these fascinating cosmic bodies, as well as helping paint the picture of how all the heavier elements in the universe are formed.
To understand the star that produced the supernova, the researchers identified the mix of elements that was thrown off right before the explosion began. Prof. Avishay Gal-Yam of the Weizmann Institute’s Particle Physics and Astrophysics Department explains that the star can be identified by the proportion of such elements as carbon, oxygen and nitrogen detected in the material ejected into space. These elements are created in the nuclear fusion that powers the stars. In our own sun, hydrogen, the lightest atom, fuses to make helium and stops there; but in the massive, hot stars, fusion continues as helium atoms unite to form heavier elements – up to iron.

Scientists believe that such stars are layered like onions: The heaviest elements, for example iron, are located in the core while the lighter ones make up the outermost layers. At the stars’ outermost edges are stellar winds that blow the material found there out to space. In stars like the one that exploded the wind is so forceful, it can throw off a mass equal to that of our sun every 10,000 years. At some point in the star’s life, the lightweight hydrogen making up its outer layer runs out, and it begins tossing out its helium, oxygen, carbon and nitrogen.
Somewhere under the surface is a layer where hydrogen, helium and the heavier elements all meet. This layer must be high enough to hold hydrogen but still hot enough to produce the extreme temperatures needed for nuclear fusion. Scientists are interested in this layer, as it is the place in which nitrogen is formed. As opposed to carbon, with six protons (three fused helium atoms), or oxygen, with eight (four heliums), nitrogen has an odd number of protons – seven. That means it must be the result of fusion between even and odd atoms, for example, three heliums and a hydrogen. So measuring the quantities of nitrogen could reveal what lies underneath the skin of such stars.
While the wind sweeps away the star’s outer layers, its core continues to amass iron until it becomes so heavy it is no longer stable. At that point, the core collapses in a sudden, violent motion, hurling the outer layers off to produce the bright supernova we observe.
Detecting the elements ejected in the stellar wind just before the explosion could only be accomplished within a small window of time – up to a day or so after the terminal blast. This is because intense radiation produced by the explosion shock strips electrons from their atoms. Telescopes equipped with spectrographs aimed at the supernova can pick up the elements’ spectra – light that is emitted when the electrons are reunited with the atoms. But they must make their observations quickly before the rapidly-expanding debris from the explosion sweep up the more tenuous remnants of the wind and erase this last trace of the dying star.
The race to observe the spectra of the supernova’s wind began with the robotic telescopes at the Palomar observatory in California, a part of the iPTF project led by Prof. Shri Kulkarni of the California Institute of Technology (Caltech). These are programmed to find transient events – sudden changes in the night sky that could be new supernovae – and alert team members about the sightings. Halfway around the world, Dr. Iair Arcavi, then a doctoral student in Gal-Yam’s group, received the notification. While researchers in the US slept, he assessed the finding, realized its significance, and contacted Dr. Assaf Horesh, then a postdoctoral fellow at Caltech (who has since joined the Weizmann team). Horesh then conducted spectroscopic observations at the Keck observatory in Hawaii, which is farther west than Palomar and could thus extend the nighttime viewing of the supernova. Acting quickly, he managed to record the emission spectra of the material thrown to the wind a mere 15 hours after the star exploded.
Working backward from the post-blast observations, Gal-Yam, Arcavi, Horesh and their colleagues assessed the recorded spectra and showed that the star that had exploded indeed had a nitrogen-rich wind, similar to those of the so-called Wolf-Rayet stars we know in our galaxy. The results of their study were published this week in Nature.This is the first time, says Gal-Yam, that this has been done. Now that the team has shown that the combination of efficient global organization and the mobilization of telescopes around the world can work to capture such fleeting events, they hope that further sightings of infant supernova explosions will be possible. Understanding how these stars live and die is important, he says, not just because it gives us a window on the workings of the universe. “All the heavier elements in the universe – those with a mass larger than that of helium – are created in the fusion furnaces of large stars and dispersed through supernova explosions. So many questions – about the origins and the relative abundance of different elements – go back to these processes taking place throughout the cosmos.”
Nature, May 22 2014.[:]