Paludisme : Weizmann (Israël), l'Irlande et l'Australie montrent comment le parasite trompe le système immunitaire
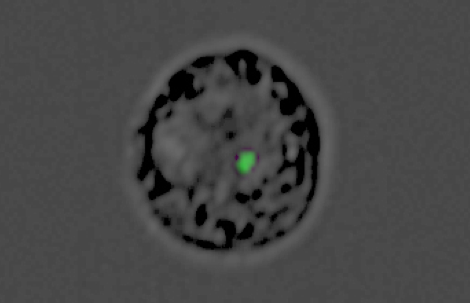
[:fr]
Le paludisme (ou malaria) est une maladie potentiellement mortelle due à des parasites transmis à l’homme par des piqûres de moustiques femelles infectés. Pour lutter contre cette maladie, les scientifiques de l’Institut Weizmann des Sciences (Israël) ont collaboré avec des laboratoires irlandais et australien pour comprendre comment ce parasite trompe notre système immunitaire aux premiers stades de l’infection. Le micro-organisme peut en effet passer les premiers stades critiques de l’infection sous les radars de nos mécanismes de défense.
La malaria est une maladie évitable et curable. Cette infection parasitaire touche 216 millions de personnes par an et en tue environ 450 000 selon le rapport de l’OMS. 90% des cas ont lieu en Afrique subsaharienne. 70% des personnes qui en décèdent sont des enfants de moins de cinq ans, ce qui représente en moyenne un enfant toutes les soixante secondes selon les statistiques de l’OMS. D’un point de vue financier, les fonds pour le contrôle et l’élimination de cette maladie s’élevaient à environ 2,7 milliards de dollars en 2016, selon l’OMS.
Cinq parasites, présents dans la piqûre de la femelle du moustique, provoquent la maladie. Parmi eux, Plasmodium (P.) falciparum est le micro-organisme qui provoque le plus de morts en Afrique, tandis que P. vivax est le plus courant. Chez l’humain, ces parasites microscopiques infectent nos globules rouges en arrivant à se propager malgré notre système immunitaire. En outre, P. falciparum présente une capacité remarquable d’adaptation aux différents médicaments et possède un cycle de vie très complexe qui empêche les vaccins de fonctionner.
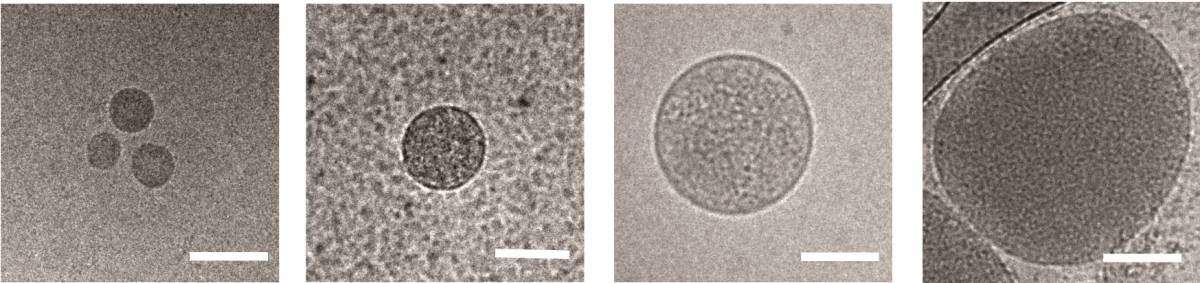
Les chercheurs de l’Institut Weizmann, en collaboration avec des équipes irlandaise et australienne, ont étudié P. falciparum pour comprendre ses mécanismes de résistance vis-à-vis de notre système immunitaire. D’après le Dr Neta Regev-Rudzki, « pour vaincre la malaria, nous devons comprendre la biologie élémentaire de P. falciparum et analyser ce qui le transforme en un tueur si redoutable ».
Dans une précédente étude, le Dr Regev-Rudzki avait déterminé la capacité des parasites à communiquer entre eux durant la période d’incubation grâce à des sacs (vésicules) remplis d’ADN. Ces vésicules sont par elles-mêmes fascinantes, car elles peuvent traverser pas moins de six membranes pour transférer l’information entre un parasite et un autre dans deux globules rouges distincts. Les micro-organismes acquièrent alors la capacité de se différentier en mâle et femelle à un moment précis et ainsi sortir du stade d’incubation.
Cette nouvelle étude montre comment ce même système de communication est utilisé par le micro-organisme pour tromper le système immunitaire. Ce processus se déroule douze heures après l’infection des globules rouges et vise cette fois-ci les monocytes, i.e. les globules blancs. Dans les faits, une protéine des globules blancs, appelée STING, est activée par P. falciparum. La première ligne de défense de notre système immunitaire se met alors à combattre le mauvais adversaire et, tandis que notre système combat un leurre, l’infection continue de se propager. Lorsque, enfin, le système immunitaire du patient détecte l’infection des globules rouges, il est souvent trop tard.
Il convient de noter que, par suppression du gène codant pour la protéine STING, les réponses immunitaires des patients sont alors bien meilleures.
Auteur : Samuel Cousin, post-doctorant à l’Institut Weizmann, pour BVST
[:en]
Global efforts to eradicate malaria are crucially dependent on scientists’ ability to outsmart the malaria parasite. And Plasmodium falciparum is notoriously clever: It is quick to develop resistance against medications and has such a complex life cycle that blocking it effectively with a vaccine has thus far proved elusive. In a new study reported in Nature Communications, researchers at the Weizmann Institute of Science, together with collaborators in Ireland and Australia, have shown that Plasmodium falciparum is even more devious than previously thought: Not only does it hide from the body’s immune defenses, it employs an active strategy to deceive the immune system.
Among transmittable diseases, malaria is second only to tuberculosis in the number of victims, putting at risk nearly half of Earth’s population. More than 200 million people become infected every year; about half a million die, most of them children under five years of age. “Malaria is one of the world’s most devastating diseases – it’s a true bane of low-income countries, where it kills a thousand young children every day,” says Dr. Neta Regev-Rudzki of Weizmann’s Biomolecular Sciences Department. “To fight malaria, we need to understand the basic biology of Plasmodium falciparum and figure out what makes it such a dangerous killer.”
Regev-Rudzki had previously discovered, in her postdoctoral studies in the laboratory of Prof. Alan Cowman at the Walter and Eliza Hall Institute of Medical Research in Melbourne, Australia, that these parasites communicate with one another while in the incubation stage in the blood. They do so by releasing sac-like nanovesicles – less than 1 micron across – that contain small segments of the parasite’s DNA. Apparently these signals help the parasites learn when it’s time to start transforming into male and female forms, both of which can be carried by mosquitoes into new hosts. This finding was all the more startling because the nanovesicles need to cross six separate membranes to communicate the message from a parasite inside one red blood cell to another.
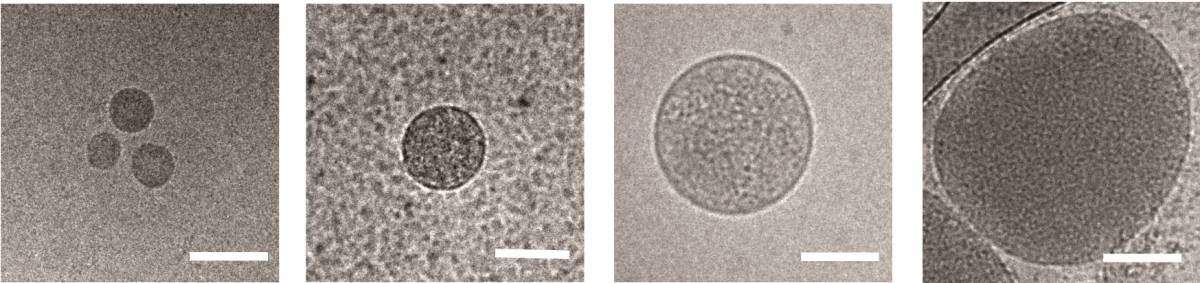
In the new study, performed in collaboration with Prof. Andrew G. Bowie of Trinity College Dublin and other researchers, Regev-Rudzki and her Weizmann team discovered that in parallel with communicating with other parasites, Plasmodium falciparum uses this same communication channel for yet another purpose: to deliver a misleading message to the infected person’s immune system. Within the first 12 hours after infecting red blood cells, the parasites send out DNA-filled nanovesicles that penetrate cells called monocytes. Normally, monocytes form the immune system’s first line of defense against foreign invasion, sensing danger from afar and alerting other immune mechanisms to mount an effective response. Naturally, the immune system dispatches its next line of defense to these cells.
But in fact, the nanovesicles have converted the monocytes into decoys. While the immune system is busy defending the organism against fake danger, the real infection proceeds inside red blood cells, allowing the parasite to multiply unhindered at dizzying speed. By the time the immune system discovers its mistake, precious time has been lost, and the infection is much more difficult to contain.
Regev-Rudzki’s team has identified a key molecular sensor, a protein called STING that becomes activated when the parasite’s nanovesicles penetrate monocytes. It is STING that delivers the fake alert to the immune system, tricking it into “thinking” that its monocytes are in danger. When the scientists “knocked out” the gene that manufactures STING, the chain of molecular reactions generating the misleading alert was interrupted.
The research team included Dr. Yifat Ofir-Birin, Paula Abou Karam and Tal Giladi of Weizmann’s Biomolecular Sciences Department; Dr. Ziv Porat of Weizmann’s Life Sciences Core Facilities Department; Dr. Xavier Sisquella, Matthew A. Pimentel, Dr. Natália G. Sampaio, Jocelyn Sietsma Penington, Dr. Andreea Waltmann, Prof. Louis Schofield, Dr. Diana S. Hansen, Prof. Anthony T. Papenfuss and Dr. Emily M. Eriksson of the Walter and Eliza Hall Institute of Medical Research, Melbourne, Australia; Dr. Lesley Cheng, Dr. Benjamin J Scicluna, Robyn A. Sharples and Prof. Andrew F. Hill of the University of Melbourne and La Trobe University in Victoria, Australia; Dr. Dympna Connolly and Prof. Bowie of Trinity College Dublin, Ireland; Dr. Dror Avni and Prof. Eli Schwartz of the Sheba Medical Center at Tel Hashomer, Israel; and Dr. Motti Gerlic of Tel Aviv University.
[:]