Weizmann and Pennsylvania U., a new model shows that the filaments in heart muscle cells don’t automatically keep the beat
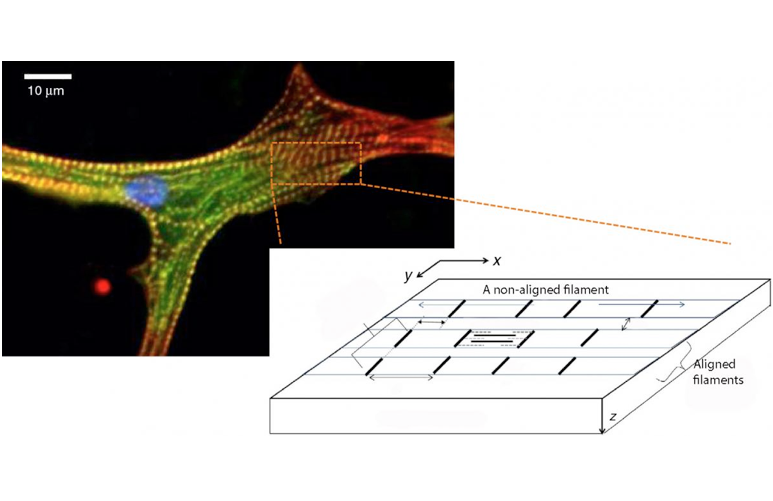
Two hearts, said Keats, can beat as one; but a study led by Weizmann Institute scientists in collaboration with researchers from the University of Pennsylvania shows that sometimes a single heart muscle cell can beat as more than two dozen. The findings provide an extremely detailed glimpse into the mechanisms behind normal and irregular heart muscle cell contractions. The study may help define the limitations of existing therapies for abnormal heartbeat and, in the future, suggest ways of designing new ones.
Each heart muscle cell consists of numerous parallel filaments comprising repeated subunits. When the heart beats, each individual filament contracts to produce muscle cell contractions.
Optimally, all the filaments should contract in a synchronized manner, thus ensuring the greatest amplitude of contraction for each muscle cell, and ultimately, the strongest and most effective beating of the entire heart. However, a new theoretical model proposed and analyzed by Prof. Samuel Safran and postdoctoral fellow Dr. Kinjal Dasbiswas of the Weizmann Institute’s Materials and Interfaces Department suggests that the filaments contract together only when their subunits, and subunit boundaries, are aligned with one another. Since such alignment usually only happens among a limited number of neighboring filaments, aligned filaments contract together as a bundle, but each such bundle contracts out of phase with others. Therefore, a heart cell does not necessarily beat as a single uniform entity; rather, the number of different beating entities in the cell depends on the bundle number, which may reach more than two dozen.
The theory, which uses the methods of statistical physics, further predicted that the alignment of the filaments in the heart muscle cell depends on the cell’s physical environment; more specifically on the elasticity of the supporting structure called the extracellular matrix. The alignment is best when this structure is not too soft and not too rigid. The prediction took into consideration various forces operating on the microscale, particularly mechanical forces that are exerted on each filament subunit by neighboring filaments via the extracellular matrix.
By assuming that only structurally aligned filaments beat together, the Weizmann theorists were able to quantitatively explain experimental findings by their collaborators from the University of Pennsylvania, Prof. Dennis Discher and Dr. Stephanie Majkut. In the experiments, these scientists had placed chick embryonic heart cells on support surfaces of varying stiffness and found that two strikingly different properties – the structural alignment of the filaments and the beating strength of the cell – depended on the rigidity of the supporting surface.
Providing a theoretical basis for these experiments, the Weizmann Institute model may help explain how filaments become aligned in heart muscle cells during embryonic development, and how their arrangement correlates with the muscle function in the adult heart. This correlation suggests that the current means of treating irregular heartbeat may be limited to a certain extent by the structural order of heart muscle filaments. But the new understanding may one day help design improved treatments for heart disease. For example, in the future, if new heart cells are grown to replace diseased ones, their growth environment may be manipulated so that their structure is well-ordered and, to paraphrase Keats, all their filaments beat as one.
Publication in Nature Communications, January 2015
Prof. Samuel Safran’s research is supported by the Gerhardt M.J. Schmidt Minerva Center on Supramolecular Architectures, which he heads; the US-Israel Binational Science Foundation; the Israel Science Foundation; Antonio and Noga Villalon, Winnetka, IL; the Clore Center for Biological Physics; the Kimmelman Center for Structural Biology; and the Kimmel Stem Cell Research Institute. Prof. Safran is the incumbent of the Fern and Manfred Steinfeld Professorial Chair. Dr. Kinjal Dasbiswas’s research is supported by a fellowship from the Council of Higher Education.