Ariel U.: Using biomimetics to replicate complex soft composite materials found in nature
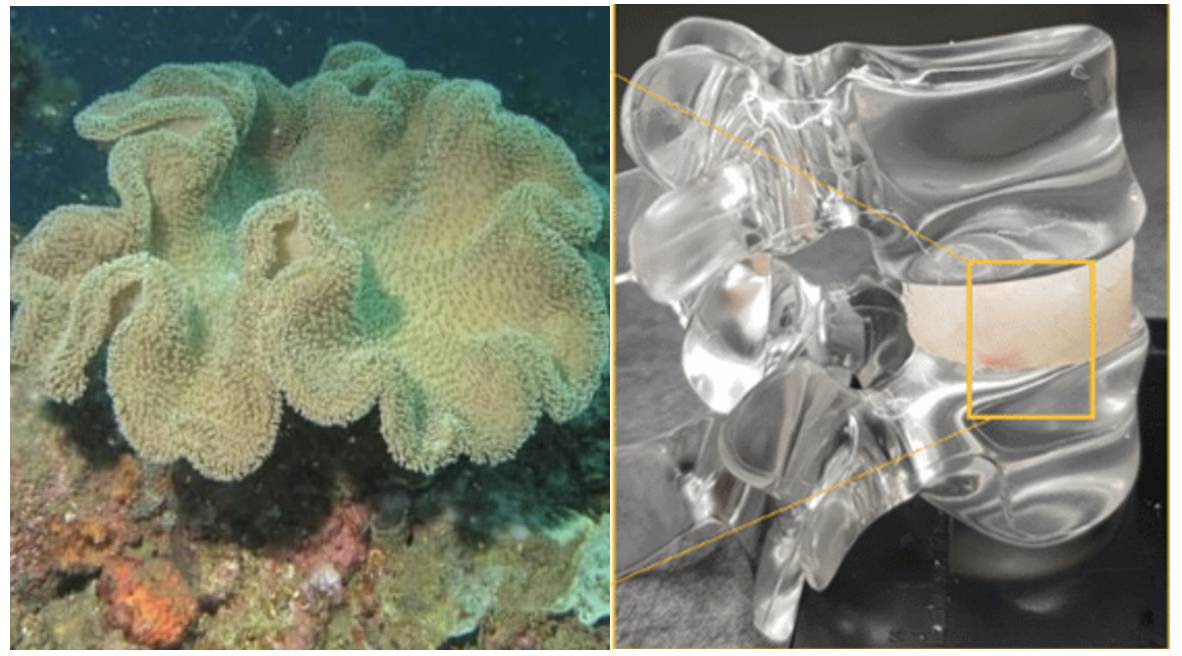
Millions of people around the world suffer from spinal disc degeneration, with only limited solutions available for relieving their pain. Dr. Mirit Sharabi, an assistant professor in the Department of Mechanical Engineering and Mechatronics at Ariel University and the Head of the Bio-inspired and Complex and Materials Mechanics (BCMM) lab, has a vision: to repair, reconstruct, or replace these damaged discs, as well as other soft tissues. Her search has taken her to the sea floor and the soft corals that abound there. “I hope that my research investigating the properties of soft composite materials will lead not only to solutions for intervertebral disc replacement but to a variety of other soft tissue transplants.”
She hopes that by understanding the nature and characteristics of natural soft composite materials at a micro-mechanical level, she will play a part in the development of new materials that will one day be used to provide “spare parts” for treating and repairing damaged tissues, like degenerated spinal discs, detached meniscus, or damaged blood vessels. Dr. Sharabi believes that as science and medicine advance, improvements in transplant tissues will also be found.
This approach, called biomimetics, emulates models, systems and elements found in nature for the purpose of solving complex human problems, which will enable better engineering of new composite materials that possess characteristics of natural materials.
She facetiously compares her research to the way the comic book character, Spiderman produces powerful spiderwebs. She creates nanofibers using high voltage electricity to build multi-hierarchical structures, similar to what is found in nature.
The same principles apply to human organs and tissues, like the meniscus, the rubbery, C-shaped cushions that serve as shock absorbers in the knee joint. Other than reattaching a torn meniscus, the alternatives available today for meniscus repair or replacement are only partially effective. To understand the mechanical behavior of soft tissues requires analyzing their structure, layer by layer. To do so, Dr. Sharabi and her team of researchers construct models of the layers of the meniscus using fiber-reinforced soft composites.
With regard to the spinal column, the special structure of spinal discs enables them to act like shock absorbers and support weights of 500 kilograms and more, and to allow movement in various directions. Dr. Sharabi explains that by trying to copy the structure of the spinal disc enables them to learn a lot about the functions they perform. “What interests me is understanding the mechanical complexities of the spinal disc. We study the minute individual layers of the tissues taken from the discs of lab animals to understand the complicated physiological properties of each part of these tissues and how they work together to enable the body’s remarkable and complex ability for movement. Then we try to replicate them by examining their structures from a mechanical and materials engineering point of view, rather than a biological orientation.”
When asked why simple plastic, rubber or silicon grafts are not suitable materials for constructing substitute disc replacements, Dr. Sharabi gives the example of the elasticity of the skin that allows it to be pulled and stretched, but eventually to return to its original shape. “Our organs and tissues are designed for specific performance. For example, look at the tremendous flexibility that gymnasts must have to bend and stretch. Materials like plastic or rubber simply do not have the properties required for that kind of combination of strength and flexibility. We are trying to recreate very specific mechanical properties found in composite materials that are capable of very specific behaviors,” says Dr. Sharabi.
The composite materials found in the tissues of our bodies are composed of three main elements: collagen, which provides strength in tissues like tendons, ligaments, and skin, etc.; elastin, which provides elasticity and flexibility; and proteoglycans, a kind of gel that provides hydration and swelling pressure to the tissues enabling them to withstand compressional forces. These three elements that are found in varying amounts in the soft tissues of our bodies enable the functioning of the various systems. As Dr. Sharabi explains, “If we use material that is too soft for a cardiovascular vessel graft, it will inflate like a balloon because it is too weak to hold the internal pressure of the blood vessel, causing it to burst.
If we use material that is too rigid, it will result in a mismatch between the vessel and the graft, which will lead to failure because the vessel will be incapable of sufficiently expanding under internal pressure. There are good reasons that every organ in our bodies behaves the way it does. This is a rather new area of research, and as a mechanical engineer, I am trying to unravel how these systems operate and why their architecture is the way it is. Together with biologists, physicians and engineers, we are working on how to replicate these tissues.”
This is where soft corals come into play. Unlike hard corals, whose skeletons are composed mainly of calcium, soft corals do not have skeletons. Collagen is what makes them sturdy. Dr. Sharabi constructs matrices of collagen fibers taken from soft corals to which hydrogel materials are added to bind them. The resulting composite is very similar to certain types of biologic tissue. However, Dr. Sharabi adds, “I am not a biologist, and I am not trying to create biological compatibility. Rather, I am interested in the mechanics of the material to understand if it behaves like the material of the spinal disc, for example.”
Dr. Sharabi and her team have assembled a construct similar to the spinal disc, mimicking its two main components. The outer layer is a fibrous structure, and the inner layer consists of a gel substance which acts as a shock absorber that transfers the motion to the outer layer. She runs computerized simulations to analyze the forces working on the composite disc, and believes the materials she is developing may have the operational capabilities of a complete disc. She and her team are now working on combining their composite outer part with a hydrogel that mimics the inner gel core. They are using a substance that is very similar to the inner nucleus pulposus gel portion of the disc, which is being developed at ORT-Braude College. Sharabi’s team is concentrating on the combination of the biomimetic annulus fibrosus and nucleus pulposus and the structural and mechanical characterization of both materials.
Furthermore, by using their new material that contains silk and collagen fibers, they have successfully replicated the behavior of several areas of discs. The results demonstrate the significance of the directionality of fibers. These experiments are conducted in the lab using computational simulations. These simulations enable examining the entire disc under complex loading modes to which the native discs are subjected. (Special equipment for advanced testing of the effects of movement on actual materials is extremely expensive and hard to come by.) The computer simulations are then compared with results from experiments of movement using cadavers. “In principle, it’s possible that the mechanical movements of discs made from our material are similar to those of actual discs,” says Dr. Sharabi.
In another project, Dr. Sharabi and her students are exploring various “morphing” life forms that are capable of changing their shapes under certain conditions. These include changes in pressure, humidity or temperature that cause seed pods, like pinecones, to open up to release their seeds. One of the factors that determine the resultant changes is the directionality of the fibers that constitute the pod and how they line up. “We carefully mimic these structures to understand how they work in nature,” explains Dr. Sharabi.
Dr. Sharabi describes herself as a very curious, creative and imaginative person who thinks out-of-the-box. She was always fascinated by nature and curious about the way things work. After breezing through high school, she gave thought to pursuing a medical career but opted instead to study biotechnology engineering at Ben Gurion University. There, she juggled between holding down several jobs to support herself and studying very hard. Upon completing her bachelor’s degree, she spent a few years working in private biomedical industries as an R&D engineer but missed the challenge of working in academic research. She decided to combine her work with completing her masters’ at Tel Aviv University after which she returned to academia and completed her PhD at Tel-Aviv University, followed by a post-doc at the Weizmann Institute before coming to Ariel University.
Source Ariel University